Functionally Stable Carbon Storage
How do we think about long-term monitoring requirements to ensure durability?
This past summer, I worked at Lowercarbon Capital with Ryan Orbuch and Kristin Ellis, researching how we can build trustworthy, credible verification systems for durable carbon removal to facilitate the scaling of the market. This is complex: we need to make quick progress on a wide variety of problems, from validation of geochemical models to a system of occupational licensing for on-site verifiers. Following up from that research and thinking, this is the first of a series of blog posts focused on what the current unmet MRV needs of the carbon removal ecosystem are, and what frameworks from other industries or new ideas might be helpful in filling these gaps.
In this post, I want to propose a framework for an under-discussed open problem in carbon removal: how do we ensure that CDR companies claiming that they’ve removed tons of CO2 from the atmosphere for 100 or 1000 years have actually done so, and that 50 or 500 years down the road that carbon won’t be re-released? Who holds the long-term liability for ensuring this, and what happens in the event of reversals?
Fundamentally, building a system on the assumption of 100-1000 year monitoring requirements and liabilities doesn’t make sense. We would need some system actor, whether that be a carbon removal company, a government body, or an insurance company, to be continuously checking up on that carbon for the next 1000 years, with a 1000-year lasting financial obligation to re-remove that carbon in the event of a reversal. What system actors will even be around that long?
There aren’t any real financial or contractual mechanisms that extend for 1000 years. Who would agree to such a thing? Carbon removal contracts are being drawn up between buyers and suppliers today, and they won’t get signed if they include continued liabilities extending hundreds of years into the future. Even being able to secure debt financing requires finite time horizons. Obviously 1000 year contracts are not real!
1000 years ago, in 1022 CE, King Robert II of France burned 13 religious heretics in Medieval Europe. The existence of carbon wouldn’t be discovered for another 750 years. Assuming we know what the world will look like in 1000 years from now is presumptuous, and is a bad premise to build the rules of a system around. In the year 3022, no one will be checking up on the tons of carbon that we remove in the next couple decades to make sure they’re still there.
We also want to make sure that there are rules around long-term responsibility for ecological risk. It’s well-known that fossil fuel companies currently evade responsibility for the environmental damage they cause by creating smaller shell companies to manage individual projects. When a well sprouts an oil leak, or when they just decide they don’t want to deal with aging infrastructure, the shell company can just declare bankruptcy, forfeiting cleanup responsibility and shedding their debts. How do we prevent this pattern from being repeated by gigaton-scale carbon removal companies?
We want rules around carbon removal liabilities to be consistent, clear to all parties, realistic, and actually followed, rather than ambiguous, naive and overly optimistic, and ignored. We definitely want to be confident that carbon actually is being stored durably, while acknowledging the need for liabilities to operate on shorter timeframes. How else can we think about this?
Landfills and Functional Stability
The exciting world of landfill management provides a useful analogy for carbon removal durability: once the landfill has filled up and the private landfill operator closes it for business, there’s now a giant pile of trash that will be sitting in that spot, basically forever. From a societal perspective, we need to make sure that it doesn’t become a threat to human health and the environment, and that *someone* assumes the responsibility for managing the pollutants and gases still coming off of it.
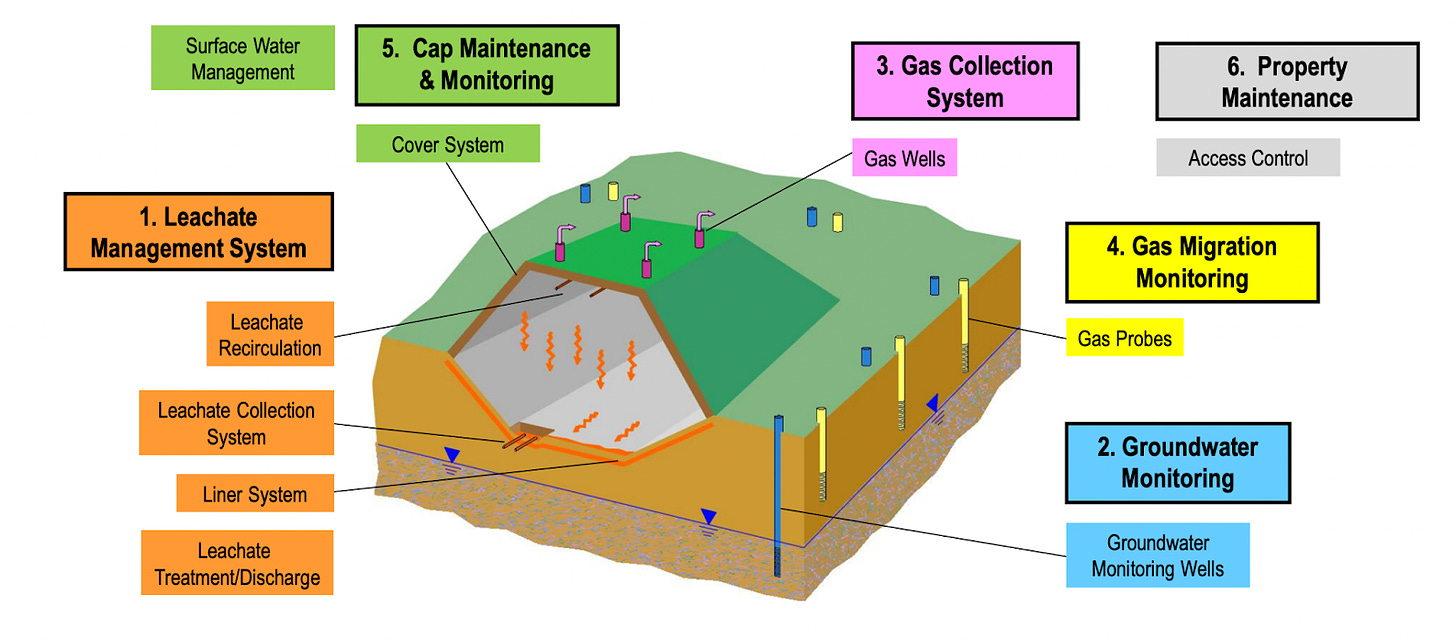
After closing, the landfill enters a “post-closure care” (PCC) period, where the private operator is legally responsible for actively collecting and treating leachate, monitoring nearby groundwater, collecting methane, and managing a cover system. This active management occurs until the landfill reaches a state known as functional stability. This occurs when “the owner or operator demonstrates that leachate [and other pollutants] no longer pose a threat to human health and the environment”, and is the point at which the private operator can stop active control and monitoring.
Functional stability doesn’t occur after some arbitrarily chosen amount of time passes—it instead requires statistical proof that the markers we care about, the ones that represent potential for environmental damage or adverse health effects, have declined sufficiently to no longer be a concern. Functional stability is an emergent property of the landfill system, and it provides a finite, usually ~30 year time horizon post-closure for landfill operators to plan around.
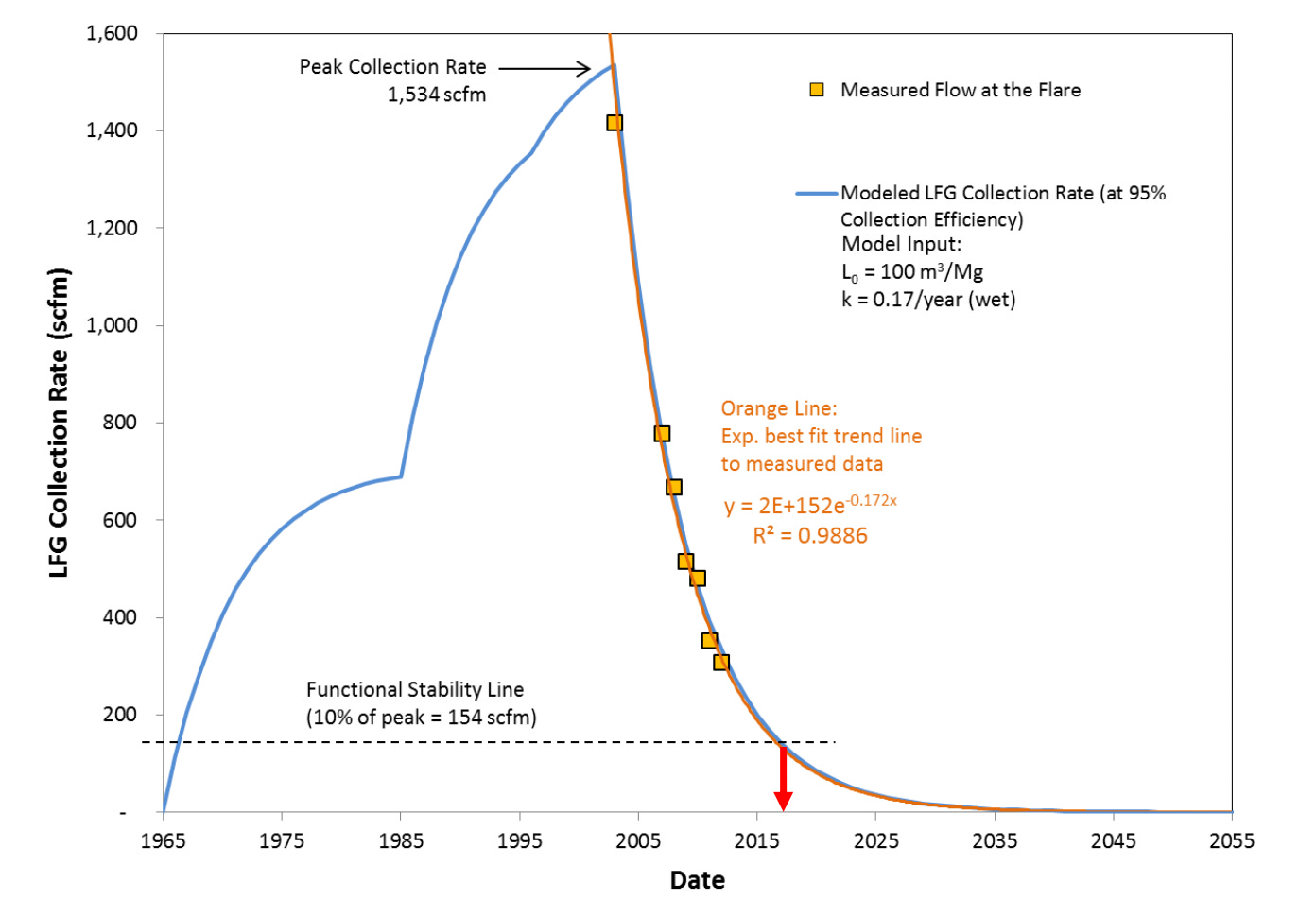
After functional stability, the landfill enters a period of “custodial care”, where a de minimis level of care is still required by whoever owns the land, mandated by deed controls or things like the Uniform Environmental Covenants Act. This mostly includes simple requirements like making sure the landfill’s cover is still in place.
In the landfill literature, functional stability is in contrast with organic stability, which only occurs when the biomass in the landfill becomes completely organically inert, long after the time when it still represents a functional risk to the environment. By using a functional stability framing instead of organic stability to determine when monitoring requirements should end, we set a more realistic timeframe for operators to plan around while also ensuring continued environmental safety.
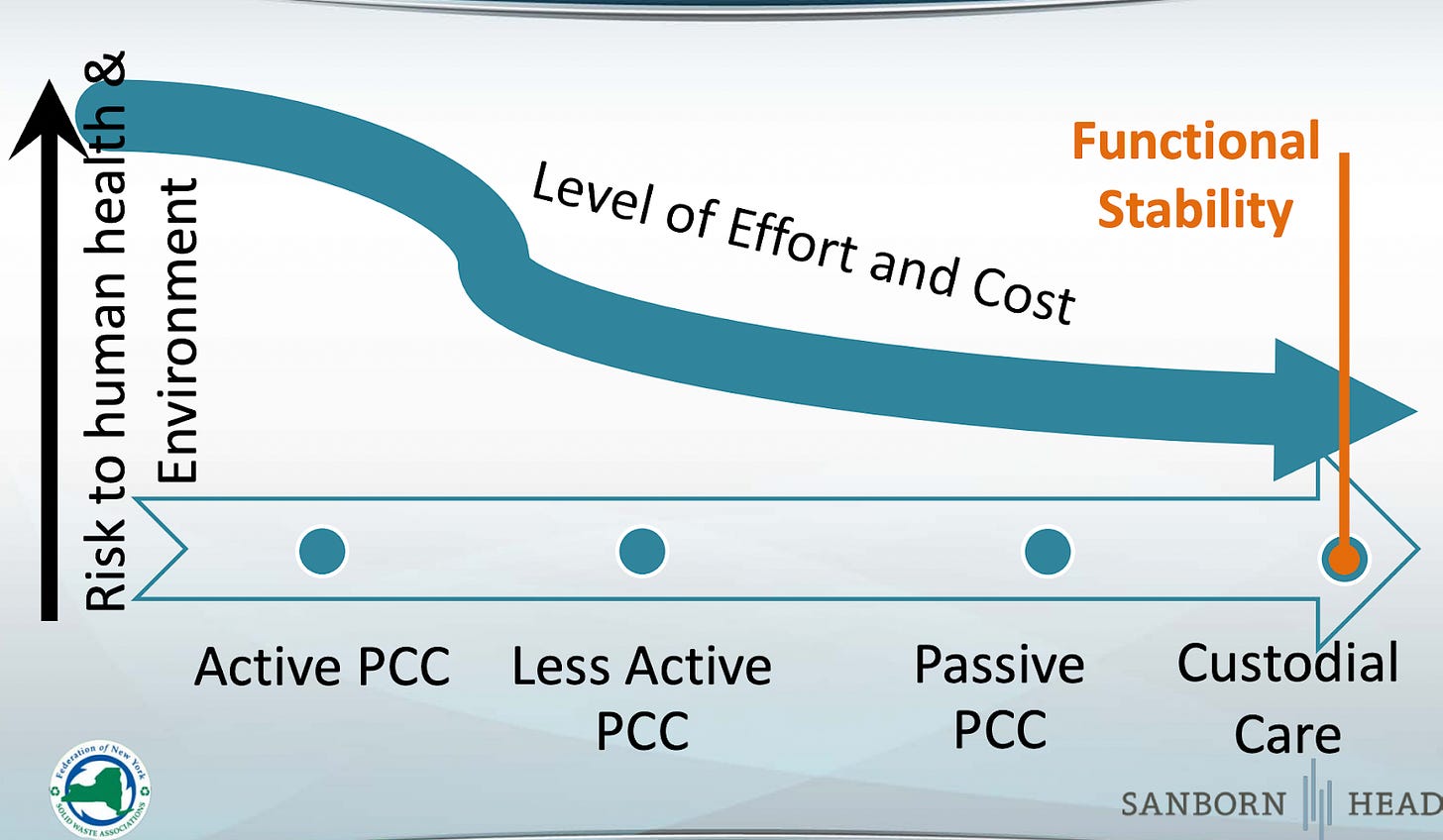
(If you’re curious, you can read more about functional stability in the landfill context here, here, and here.)
Durable CDR and Functional Stability
Let’s now draw the functional stability analogy to carbon removal. We can say that a ton of carbon removed has reached functional stability once we have sufficient site data or other evidence to suggest that the risk of premature reversal or continued ecological damage is sufficiently low, in the absence of active control systems. Then, we declare the stored carbon to be functionally stable, and the project developer no longer needs to keep monitoring. The project has fulfilled their responsibility for that ton and no longer has a continued liability.
Just like in the landfill case, the point at which tons reach functional stability is an emergent property of the geophysical system where the carbon is stored. We’re not making up an arbitrary endpoint when monitoring ends, just for the sake of not having to deal with long time horizons. The goal here, when done correctly, is to come up with a set of criteria that can be measured, modeled, or otherwise known about the storage reservoir that would indicate we have sufficient confidence in safe, continued storage to wind down liabilities and monitoring. It’s up to us, as the CDR community, to rigorously decide on what these functional stability criteria should be for each pathway.
For each carbon storage reservoir, it’s useful and directly actionable for earth system scientists to be writing papers and having this more specific debate about what the right parameters and criteria that should define functional stability are. That is, given what we know about the properties and dynamics of a storage reservoir, what site data should we accept as sufficient evidence that reversal risk is low enough to end monitoring?
By having this discussion and coming to consensus, we can make monitoring requirements for projects clear, consistent, and science-based. It allows us to be realistic about the contract lengths we can realistically impose as well as the longevity of the institutions involved, while also not letting CDR projects off the hook for ensuring durability. This definition then can live in a lot of places—from measurement protocols and current contracts with buyers, to eventual government regulations and future CDR insurance and surety policies.
Examples and Complexities
Before we get into any specific pathways, the picture below represents a very high-level timeline for how carbon removal works. There is 1) some action or intervention taken that (maybe after some time delay), results in 2) tons of CO2 being removed from the atmosphere, which after some time and wrangling will 3) get to functional stability, where we are confident in the continued storage of those tons. There is some time delay between each of these steps, and adding up these delays gives us the total time that a project is on the hook for monitoring that ton.
This creates a new frame for the emerging conversations about quantification of carbon removals. Instead of asking the question, “How many net tons did a project remove?”, we can now more appropriately ask “How many net tons did a project bring to functional stability?”. Only when a project proves that it has fully wrangled the tons into durable storage, fulfilling their responsibility, should they really get full credit for their removal.
Part of what makes a durable carbon removal pathway “good” is that it reaches functional stability on a relatively short timeframe, so that we can be done with removed tons faster and we don’t have to deal with long-term complications. In some sense, the ethos of durable carbon removal is that we can do some action today with confidence that it will take carbon out of the atmosphere long term, without requiring continued active effort over the long-term.
To better illustrate some of the nuances of how this concept works, let’s go through three examples of carbon getting to functional stability across a variety of durable carbon removal pathways.
Example 1: Direct Air Capture + Injection
Consider a ton of carbon removed from the atmosphere via DAC, and then injected underground in some sort of storage well. By our three box diagram above, the removal action (running the DAC machine) and the tons coming out of the atmosphere occur at the same time. However, there is a time delay between when the CO2 comes out of the atmosphere and when long-term monitoring can stop; namely, we have to wait for the carbon to stabilize sufficiently within the well.
In trying to define functional stability for this system, we need to look at the specific properties of the storage reservoir. Are we injecting into a sedimentary basin, where the CO2 will initially only be physically trapped underneath an impermeable caprock, or into a basalt formation, where the CO2 will quickly mineralize?
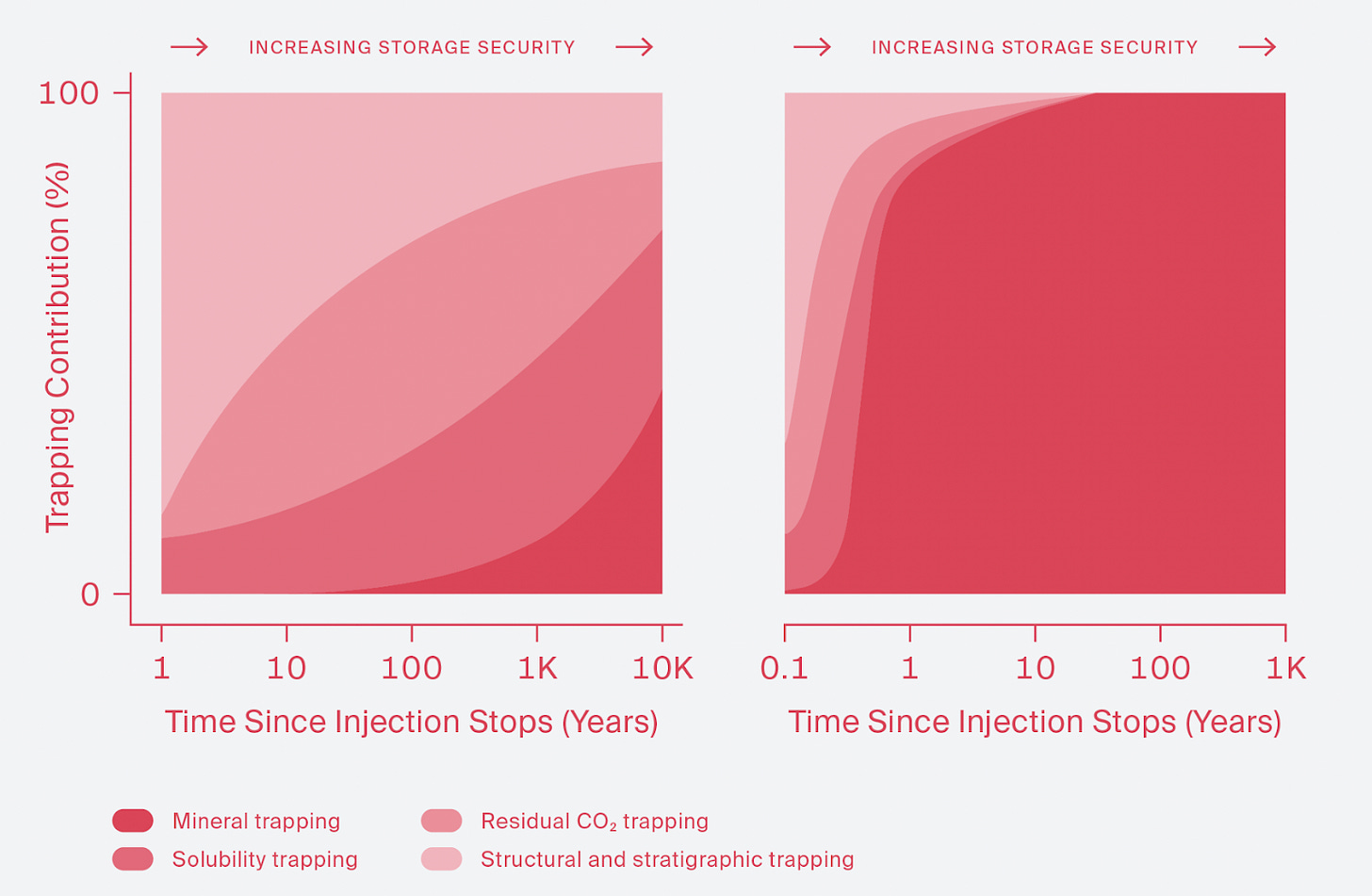
The above image from the CDR Primer compares sedimentary wells (left) to basalt wells (right) across time after injection: notice that CO2 in basalt formations almost completely mineralizes after only 5-10 years, whereas in sedimentary reservoirs the majority of trapping even after 50 years is still done by weaker, less stable mechanisms like structural and residual trapping. Clearly, CO2 in the basalt formation will reach functional stability faster than CO2 in the sedimentary basin.
But rather than choosing an arbitrary vertical line on each graph and setting that as the monitoring time horizon, we still need to define measurable functional stability criteria for each storage reservoir, based on how we evaluate the instantaneous probability of reversals over time in each system. We pretend that durability is just a number, like 100 or 500 years, after which all of the carbon will immediately be reversed. But really it’s a probability distribution representing the likelihood at each moment in time that reversals will occur. Functional stability represents the point at which the probability of reversal is sufficiently low that we can pack up our bags and stop monitoring
.
The EPA’s requirements for the long-term monitoring of Class VI (sedimentary) wells specify: “The owner or operator must continue to monitor the site for 50 years following the cessation of injection, or for an approved alternative timeframe, until it can be demonstrated that no additional monitoring is needed to ensure that the project does not pose an endangerment to USDWs [underground sources of drinking water]”. The alternative timeline resembles functional stability pretty closely, and more closely matches requirements to what is actually geophysically happening in the well.
Example 2: Ocean Alkalinity Enhancement via Mineral Dissolution
Now, consider a carbon removal pathway in which solid ultramafic minerals are added to the surface ocean, where they will dissolve and increase ocean alkalinity. CO2 will thus be removed from the surface ocean, and because the surface ocean and atmosphere are in equilibrium, the ocean will uptake CO2 from the atmosphere.
Here, the removal action is adding the solid minerals to the ocean. The delay to tons coming out of the atmosphere in this case is substantial: we need to wait for the minerals to dissolve, and then we need to wait for the air-sea flux to respond to depleted CO2 in the ocean.
What about functional stability? At what point can we stop monitoring this system, with confidence that durable carbon removal has been achieved?
Part of the issue is that the alkalinity signal will get diluted so quickly, so “monitoring” the stored CO2 for more than a couple of hours becomes impossible. That said, if we’re going to scale OAE to gigatons, we need to understand under what conditions we would lose our increase in alkalinity, perhaps through secondary carbonate precipitation. What happens if secondary carbonate precipitation occurs several weeks after mineral addition or dissolution? Through some combination of modeling, clever measurements, and characterization of particular deployment sites, we still need to come to some answer for what markers should be satisfactory to indicate functionally stable carbon storage.
Lastly, mineral dissolution OAE approaches deployed at scale could potentially lead to negative ecological impacts, whose effects may not be fully visible for long after we feel confident that the carbon itself won’t undergo reversals. The upshot is that there still may be cases where we still want to require monitoring for ecological reasons or other side effects, even if we feel good about the carbon storage itself. Functional stability only is reached once we feel confident enough to wind down monitoring all together.
Example 3: Macroalgae Sinking
As a final example, consider a carbon removal project that grows kelp on the open ocean and sinks it to the seafloor. Once there, most of the seaweed will be respired and converted back to CO2, but the CO2 won’t be able to reach the atmosphere until the ocean’s overturning circulation pushes that parcel of deep sea water back to the surface, which depending on where the sinking occurred won’t happen for another 600-1000 years.
Here, the functional stability criteria probably look something like 1) making sure the seaweed successfully sinks to the seafloor without being eaten and remineralized somewhere along the way, and 2) ensuring that ecological effects like deep sea anoxia or surface ocean planktonic composition are controlled and kept within safe levels.
The one subtlety here is that for kelp sinking, functional stability doesn’t actually mean that the CO2 will stay out of the air *essentially forever*, like it does in the DAC+injection and OAE cases. The overturning circulation will eventually bring the CO2 back to the surface, but once we’re confident about functional stability being reached by the kelp system, we are claiming that there won’t be any pre-mature reversals, before a bounded, calculable, and sufficiently long period of time (at least many hundreds of years in this case). Thus, we can stop monitoring those tons, and feel assured that they’ll stay removed for the expected, high durability length of time.
Generalizing now beyond these examples, to make functional stability work, we need to answer the following questions for each pathway:
What are the conditions of continued carbon storage for the given storage reservoir?
What measurements, rigorous modeling results, or other data are sufficient to convince us that removed tons have reached these conditions, and that these conditions will not change?
How long do these functional stability conditions guarantee us that tons will stay out of the atmosphere?
If there’s a lot of uncertainty in our answers to these questions, this represents a fundamental lack of understanding about the pathway as a whole, about whether it even works as a durable carbon removal pathway. We need to do more science to figure out how the storage reservoir works before scaling. This differs from the quantification uncertainty recently discussed by CarbonPlan and Frontier that has instead to do with errors bars for “how much” was removed, where some uncertainty for open systems is expected and often irreducible.
When Should Credits Get Issued?
One last subtlety here: if under this frame, a project doesn’t finish their responsibility for a delivery until after the carbon reaches functional stability, when should that project officially get credit for that delivery? When should they get paid for it? If they get paid for the delivery before it reaches functional stability, how do we ensure that the project still fulfills monitoring requirements and re-removes tons to make up for pre-functional stability reversals?
It doesn’t really make practical sense to force projects to get their tons to functional stability before getting paid for them—if the delay is even just 5 or 10 years, waiting that long for additional capital to begin the next deployment will prevent them from getting to any scale at all.
So how can we still ensure that companies still do monitoring until functional stability, and that reversals are made up for?
Going back to the landfill analogy, there are insurance mechanisms in place called “surety bonds” that private landfill operators are required by law to purchase. Basically, the insurance company that sells the surety bond takes on the risk that the private operator doesn’t complete post-closure care, being forced to pay out and ensure the successful completion of the process if the landfill operator goes bankrupt or evades responsibility.
So maybe you can pay companies upon first removal but before functional stability, as long as there are legal mechanisms in place that ensure that they, or barring that at least *someone*, gets the correct number of tons to functional stability.
More thinking is definitely still needed on this framework and on the contractual and legal structures that would implement functional stability practically. Building this system from scratch won’t be easy, and there are lots of non-obvious decisions to make about what criteria should define functional stability in each carbon storage reservoir, what commitments and liabilities are actually reasonable for projects, and how to take into account uncertain ecological risks. But these are important conversations to get going so that when large scale carbon removal deployments start coming online, we have good mechanisms in place to realistically ensure that carbon is durably stored.
Thanks to Dai Ellis and Ryan Orbuch for feedback on this and earlier drafts.